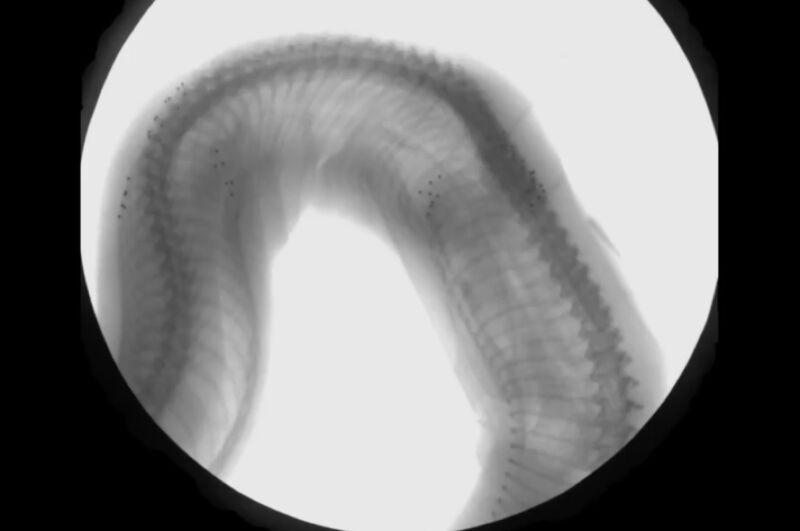
Watching a boa constrictor capture and consume its prey is quite something. First, the snake strikes and latches onto the prey with its teeth, then it coils its body tightly around the poor creature and slowly squeezes the life from it. The constrictor cuts off blood flow to the heart and brain. Then the boa unhinges its jaw and swallows the prey whole. The boa uses its muscles to move its prey down the length of its body to the stomach, where the unlucky varmint is digested over the next four to six days.
Boa constrictors mostly consume various medium-sized rodents, lizards, and birds. They have also been known to chow down on even larger prey, including monkeys, wild pigs, and ocelots. Regardless of what's on the menu, how do the snakes still manage to breathe as they crush an animal to death, since that constriction also uncomfortably squeezes the boas' own ribs? Unlike mammals (including humans), boa constrictors don’t have a separate diaphragm. They rely entirely on the motion of their ribs to breathe.
Biologists at Brown University and Dickinson College conducted a series of experiments to find out more, and they described their results in a new paper published in the Journal of Experimental Biology. Boa constrictors, they discovered, have a remarkable ability to selectively use different sections of their rib cage for breathing during constriction. The reptiles essentially use the far end of their lungs as a bellows to pull in air whenever the ribs closer to the head are obstructed.
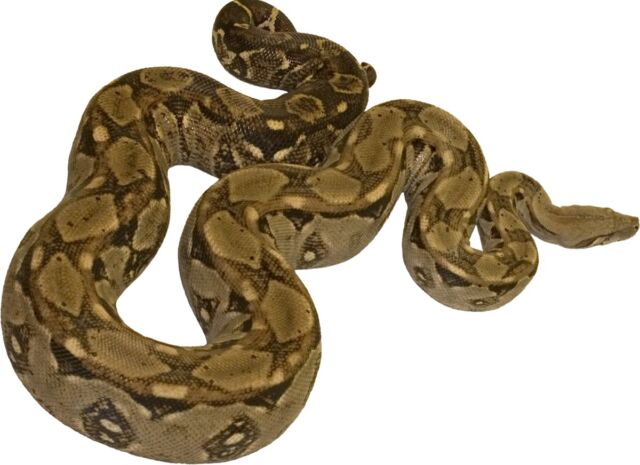
The team used a combination of techniques for their study to gather critical data on airflow, muscle activation, and rib motion in vivo. All but one of the snakes used in the experiments were born in captivity, bred from boa constrictors captured in the wild in Belize. The sole outlier was purchased from a reputable reptile breeder, per the authors.
Co-author John Capano of Brown University performed the x-ray experiments, using a technique known as XROMM (X-ray reconstruction of moving morphology) to create X-ray movies of the snakes. He also took CT scans and used that data to reconstruct the rib and vertebrae movements in a computer model. Capano first attached tiny metal markers to two ribs in each of three adult female boa constrictors. One marker was placed about a third of the way down the body length, and the other was placed halfway down.
Next, Capano placed blood pressure cuffs over the ribs in those two spots, and gradually increased the pressure to immobilize the snakes—essentially simulating what would occur as they crushed their prey. Some snakes didn't seem to mind the cuff, per Capano, while others hissed. The latter response proved ideal for the experiments, since hissing requires the snakes to fill their lungs full of air. Hence, the hissing snakes produced the biggest breaths that Capano was able to measure.
The team used pneumotachography (often used to study sleep apnea and related disorders in humans) to monitor the airflow in five boa constrictors, fabricating small lightweight masks for the snakes out of plastic bottles. The snake breaths passed through with a PVC tube containing a fine metal mesh to provide some resistance to the airflow. The pressure difference over that fixed resistance yields the flow rate.
The authors acknowledged that these results were inconsistent, mostly because the snakes kept taking off their masks. (Even humans find the procedure uncomfortable, so one can hardly blame the snakes.) However, the method provided reliable data on pressure variation and volume changes as the snakes breathed in and out, and the biologists visually confirmed that data in the X-ray videos in several cases.
Capano's co-authors at Dickinson College, Scott Boback and Charles Zwemer, took on the task of determining whether the snakes were capable of specific patterns of muscle activation. This was done by recording the nerve signals that controlled the rib muscles as pressure was being applied with the cuffs on one adult female boa and one adult male boa, using a technique called electromyography. Finally, Boback serendipitously managed to capture one snake mid-meal using a GoPro camera. He found that there were no nerve signals in the constricted muscle at all; rather, the snake activated a different set of ribs further down its body to continue breathing.
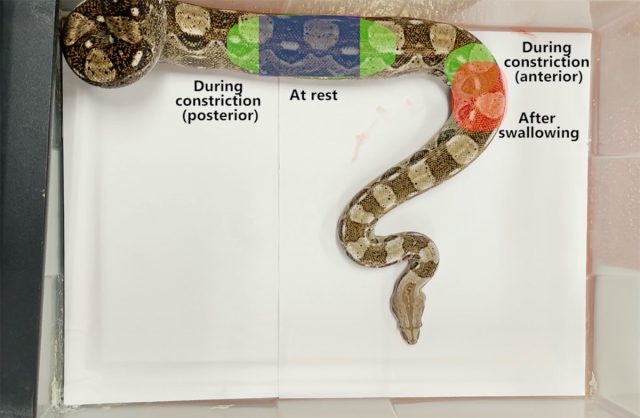
"We found multiple lines of evidence in support of our hypothesis that boa constrictors actively modulate the trunk segments and ribs used for lung ventilation, in response to hindered rib motions," the authors wrote. When pressure was applied to the cuff a third of the length down the body, the snakes responded by activating the ribs further back to breathe. The snakes swung them backward and tipped them up to get air into their lungs. When pressure was applied further down the body, toward the far end of the lung, the snakes activated the ribs closer to the head to breathe.
Based on their findings, the authors suggest that the ability to constrict or ingest large prey would not have emerged unless the snakes had first gained the ability to sustain the high metabolic costs and regulate their breathing while doing so. Thus, this "modular lung ventilation" trait likely co-evolved with those other two traits. The ability to selectively activate different segments of the ribs in order to breathe while consuming large prey would also help conserve energy.
"Without such a mechanism, early snakes would have been unable to circumvent the mechanical and physiological constraints each behavior subsequently produced," Capano et al. concluded. "This interplay of traits would have enabled early snakes to subdue and ingest a wider variety of prey species and expand their ecological roles beyond those of other elongate vertebrates, facilitating the remarkable [diversity] of snakes we observe today."
DOI: Journal of Experimental Biology, 2022. 10.1242/jeb.243119 (About DOIs).
reader comments
33